Atmospheric bores-SSEC instrument provides detailed look at boundary layer phenomenon
A new study by researchers at the University of Wisconsin-Madison Space Science and Engineering Center (SSEC) and the Department of Atmospheric and Oceanic Sciences (AOS) demonstrates the promise of ground-based atmospheric measurements in producing better severe weather forecasts.
Each summer, thunderstorms erupt across the US Great Plains and other areas. There is a predictable order to this type of storm as it sweeps across the landscape: strong winds typically followed by rain. This process, known as a gust front, is the result of different air masses clashing and mixing at the leading edge of the storm. Scientists want to learn more about these dynamics in order to improve forecasts.
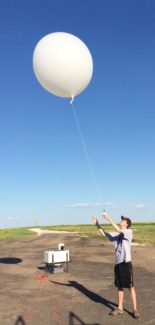
UW-Madison AOS grad student David Loveless launches a radiosonde attached to a weather balloon during a PECAN observation period on June 09, 2015. Photo credit: Lee Cronce SSEC
In certain cases, this tumbling air can trigger a phenomenon known as an atmospheric bore, a gravity-wave structure, or ripple, that travels ahead of the gust front and is thought to help strengthen storms through increased convection. This occurs in the boundary layer, the region of the atmosphere just above the Earth’s surface.
Because there are limited case studies on atmospheric bores and how they form, SSEC and AOS researchers set out to profile eight separate instances of the phenomenon in order to compare how they change the boundary layer. The results of their study were published in the April 2019 issue of Monthly Weather Review.
The study analyzed data from the Plains Elevated Convection at Night (PECAN) experiment, a massive field campaign conducted during the summer of 2015. During PECAN, researchers from institutes around the country canvassed the Great Plains states to collect data on severe storms with an array of ground-based instruments.
Among them, the SSEC Portable Atmospheric Research Laboratory (SPARC) gathered high resolution data of approaching thunderstorms and bores using the Atmospheric Emitted Radiance Interferometer (AERI). The AERI’s strength is the precision with which it uses infrared light to precisely measure temperature, wind and moisture profiles throughout much of the boundary layer and beyond.
“Using the AERI data we were able to look more deeply into the effects of bores and provide some context to the atmosphere before and after a bore passes through,” says David Loveless, UW-Madison AOS graduate research student.
The AERI profiles were compared with other atmospheric measurements taken at the same time using different instrumentation like radiosondes, radar, Doppler lidar, and the Collaborative Lower Atmosphere Profiling System (CLAMPS) developed by the University of Oklahoma and the National Severe Storms Laboratory. These helped to create a comprehensive scan of the varying conditions in which the bores formed. Most notably, Loveless says the results show that upward motion caused by atmospheric bores helps dissipate the capping inversion, allowing air to rise and develop clouds. In doing so, the surrounding atmosphere becomes more unstable, making it easier for thunderstorms to form.
“Across eight case studies we showed that bores, on average, tend to increase the likelihood of convection and thus storm development behind it,” says Loveless. “This is due to a reduction in static stability, as well a reduction in the levels of free convection.”
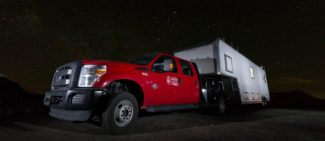
The Space Science and Engineering Center (SSEC) Portable Atmospheric Research Center (SPARC) is a specially designed vehicle outfitted with instruments that make ground-based measurements used for studying the atmosphere. Photo credit: Tim Wagner
Co-author Tim Wagner, a researcher at SSEC, says case studies like these show the power of ground-based atmospheric measurements and how they can eventually help improve predictions for severe weather. When used in tandem with measurements from instruments like the CLAMPS, scientists can see rapidly changing boundary layer conditions. According to Wagner, detecting atmospheric bores using the existing network of instruments is a difficult task, but using platforms like the AERI and CLAMPS allows for a greater likelihood of detecting them while gaining a high resolution look at their effects.
Using the instruments aboard the SPARC and CLAMPS, they can collect data 24 hours a day, and as frequently as once every 30 seconds. Another instrument (specific to SPARC), is the High Spectral Resolution Lidar that is used to measure winds and detect specific pollution particles found in the boundary layer, all the way to the edge of Earth’s atmosphere.
“Going forward, we will have to rethink how boundary layer information is assimilated into weather models since so much of it right now is based on the twice daily weather balloon launches,” says Wagner. “With the level of detail we get from AERI and CLAMPS data, we can start to fill in the gaps between weather balloon launches and pair that important boundary layer information with that collected by weather satellites.”
Over the last ten years, the SPARC and CLAMPS instruments have demonstrated a wide-range of capabilities throughout several field campaigns. Thus far, they have been used for severe weather detection, satellite validation, air quality monitoring and various process studies. This coming field season the team will deploy the SPARC as part of the CHEESEHEAD campaign, a study designed to understand the impacts terrain, trees and other plant life can have on the weather.
“Our goal is to expand the use of these boundary layer measuring tools like the SPARC and CLAMPS and to create permanent field sites for them,” says Wagner. “We want to broaden our collaborations and further demonstrate the potential benefits of incorporating these data into existing processes to forecast severe weather, especially in areas that are vulnerable to dangerous storms.”
This work was supported by the National Science Foundation.